Ocean Salinity and the Global Water Cycle
Water evaporating from the ocean surface sustains precipitation on land. This ocean-to-land moisture transport leaves an imprint on sea surface salinity (SSS), which can be measured by in situ observations and emerging remote sensing capabilities. This raises the question whether variations in SSS can provide insight into understanding and predicting terrestrial precipitation. Furthermore, the long-term trend of SSS reveals an intensification of the global hydrological cycle due to human-induced climate change. In a series of studies, we explored the role of ocean salinity for understanding the global water cycle (Li et al. 2016b, Li et al. 2018, Liang et al. 2020, Rathore et al. 2020, Ummenhofer et al. 2021), including variations in terrestrial precipitation, as well as the utility of SSS for improved predictions (e.g., Li et al. 2016a, 2022, Rathore et al. 2021)
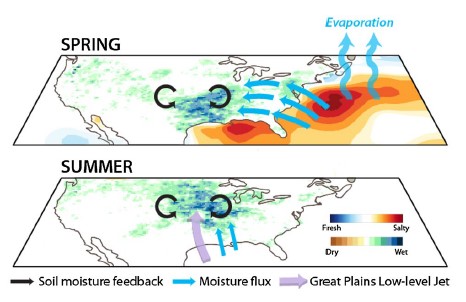
Ocean salinity as a measure of an intensifying water cycle
Across the Indo-Pacific region, rapid increases in surface temperatures, ocean heat content and concomitant hydrological changes have implications for sea level rise, ocean circulation and regional freshwater availability. In Ummenhofer et al. (2021), we synthesize evidence from multiple data sources to elucidate whether the observed heat and freshwater changes in the Indian Ocean represent an intensification of the hydrological cycle, as expected in a warming world. At the basin scale, 20th century warming trends can be unequivocally attributed to human-induced climate change. Changes since 1980, however, appear dominated by multidecadal variability associated with the Interdecadal Pacific oscillation, manifested as shifts in the Walker circulation and a corresponding reorganization of the Indo-Pacific heat and freshwater balance. Such variability, coupled with regional-scale trends, a short observational record and climate model uncertainties, makes it difficult to assess whether contemporary changes represent an anthropogenically forced transformation of the hydrological cycle. Future work must, therefore, focus on maintaining and expanding observing systems of remotely sensed and in situ observations, as well as extending and integrating coral proxy networks. Improved climate model simulations of the Maritime Continent region and its intricate exchange between the Pacific and Indian oceans are further necessary to quantify and attribute Indo-Pacific hydrological changes.
The Amazon river basin receives ~2000 mm of precipitation annually and contributes ~17% of global river freshwater input to the oceans; its hydroclimatic variations can exert profound impacts on the marine ecosystem in the Amazon plume region and have potential far reaching influences on hydroclimate over the tropical Atlantic. In Liang et al. (2020), we show that an amplified seasonal cycle of Amazonia precipitation, represented by the annual difference between maximum and minimum values, during the period 1979–2018, leads to enhanced seasonality in both Amazon river discharge and plume ocean salinity. An atmospheric moisture budget analysis shows that these enhanced seasonal cycles are associated with similar amplifications in the atmospheric vertical and horizontal moisture advections. Hierarchical sensitivity experiments using global climate models quantify the relationships of this enhanced seasonality. The results suggest that an intensified hydroclimatological cycle may develop in the Amazonia atmosphere-land-ocean coupled system, favoring more extreme terrestrial and marine conditions.
Ocean salinity as a tool to understand and predict rainfall variability on land
Li et al. (2016a) provides evidence that springtime SSS in the subtropical North Atlantic can be used as a predictor of terrestrial precipitation during the subsequent summer monsoon in Africa. Specifically, increased springtime SSS in the subtropical North Atlantic tends to be followed by enhanced monsoon-season precipitation in the African Sahel. In the spring, high SSS is associated with stronger moisture flux divergence from the subtropical oceans, which converges over the African Sahel and elevates local soil moisture. From spring to summer, the initial water cycling signal is preserved, amplified, and manifests in excessive precipitation. Because of the physical connection between salinity, ocean-to-land moisture transport, and local soil moisture feedback, seasonal forecasts of Sahel precipitation can be improved by incorporating SSS into prediction models. Thus, expanded monitoring of ocean salinity should contribute to more skillful predictions of precipitation in vulnerable subtropical regions, such as the Sahel.
Moisture originating from the subtropical North Atlantic feeds precipitation throughout the Western Hemisphere. This ocean-to-land moisture transport leaves its imprint on SSS, enabling SSS over the subtropical oceans to be used as an indicator of terrestrial precipitation. Li et al. (2016b) demonstrates that springtime SSS over the northwestern portion of the subtropical North Atlantic significantly correlates with summertime precipitation over the U.S. Midwest. The linkage between springtime SSS and the Midwest summer precipitation is established through ocean-to-land moisture transport followed by a soil moisture feedback over the southern U.S. In the spring, high SSS over the northwestern subtropical Atlantic coincides with a local increase in moisture flux divergence. The moisture flux is then directed toward and converges over the southern U.S., which experiences increased precipitation and soil moisture. The increased soil moisture influences the regional water cycle both thermodynamically and dynamically, leading to excessive summer precipitation in the Midwest, as detailed in the study. Owing to these robust physical linkages, the springtime SSS outweighs the leading SST modes in predicting the Midwest summer precipitation and significantly improves rainfall prediction in this region.
The role of the oceanic water cycle in the record-breaking 2015 warm-season precipitation in the U.S. is analyzed in Li et al. (2018). The extreme precipitation started in the southern U.S. in the spring and propagated northward to the Midwest in the summer of 2015. This seasonal evolution of precipitation anomalies represents a typical mode of variability of U.S. warm-season precipitation and is linked to moisture export from the subtropical North Atlantic. The North Atlantic moisture flux interacts with local soil moisture which enables enhanced moisture to be drawn in from the Gulf of Mexico in the summer. Further analysis shows that the relationship between Midwest U.S. rainfall and the North Atlantic has become more significant in recent decades, indicating an increased likelihood of extremes like the 2015 case. Indeed, two record-high warm-season precipitation events (i.e., 1993 and 2008) both occurred in the more recent decades of the 66 year analysis period. The export of water from the North Atlantic leaves a marked surface salinity signature. The SSS signature appeared in the spring preceding all three extreme precipitation events, i.e. a saltier-than-normal subtropical North Atlantic in spring followed by extreme Midwest precipitation in summer. Thus, our study suggests that pre-season salinity patterns can be used for improved seasonal prediction of extreme precipitation in the U.S. Midwest, as is further demonstrated in Li et al. (2022): combined with soil moisture feedbacks bridging the springtime North Atlantic salinity, the SSS-based statistical prediction model improves U.S. Midwest heavy rainfall forecasts by 92%, complementing existing sea surface temperature-based frameworks.
For Australia, Rathore et al. (2020) demonstrates that SSS variability can also be used as a measure of terrestrial precipitation on interseasonal to interannual time scales and for determining moisture sources. Seasonal composites during El Niño-Southern Oscillation/Indian Ocean Dipole (ENSO/IOD) events are used to understand the variations of moisture transport and precipitation over Australia, and their association with SSS variability. During co-occurring La Niña and negative IOD events, salty anomalies around the Maritime Continent indicate freshwater export and are associated with a significant moisture transport that converges over Australia to create anomalous wet conditions. The significant pattern correlation between the moisture flux divergence and SSS anomaly during the ENSO/IOD events highlights the associated ocean-atmosphere coupling. A case study of the 2010/11 Brisbane flood demonstrates that changes in SSS occur before the peak of ENSO/IOD events. This raises the prospect that tracking of SSS variability could aid Australian rainfall predictions. As such, Rathore et al. (2021) uses SSS as an additional precursor for improving the prediction of austral summer rainfall over northeastern Australia. Using random-forest regression analysis, it is shown that local soil moisture, ENSO, and Indo-Pacific warm pool SSS are the most important precursors for northeast Australian rainfall. The improved Australian rainfall prediction when including SSS from the prior season suggests that sustained observations of SSS can aid with anticipating water cycle variations in Australia.
Li L, Schmitt RW, Ummenhofer CC, and Karnauskas KB. (2016). North Atlantic salinity as a predictor of Sahel rainfall. Science Advances, 2, doi:10.1126/sciadv.1501588. Reprint
Li L, Schmitt RW, Ummenhofer CC, and Karnauskas KB. (2016). Implications of North Atlantic sea surface salinity for summer precipitation over the US Midwest: Mechanisms and predictive value. Journal of Climate, 29, 3143-3159. Reprint
Li L, Schmitt RW, and Ummenhofer CC. (2018). The role of the subtropical North Atlantic water cycle in recent US extreme precipitation events. Climate Dynamics, 50, 1291-1305. Reprint
Li L, Schmitt RW, and Ummenhofer CC. (2022). Skillful long-lead prediction of summertime heavy rainfall in the US Midwest from sea surface salinity. Geophysical Research Letters, doi:10.1029/2022GL098554. Reprint
Liang Y-C, Lo M-H, Lan C-W, Seo H, Ummenhofer CC, Yeager S, Wu R-J, and Steffen JD (2020). Amplified seasonal cycle in hydroclimate over the Amazon river basin and its plume region in the Atlantic. Nature Communications, doi:10.1038/s41467-020-18187-0. Reprint
Rathore S, Bindoff NL, Ummenhofer CC, Phillips HE, and Feng M. (2020). Near-surface salinity reveals the oceanic sources of moisture for Australian precipitation through atmospheric moisture transport. Journal of Climate, 33, 6707-6730. Reprint
Rathore S, Bindoff NL, Ummenhofer CC, Phillips HE, Feng M, and Mishra M. (2021). Improving Australian rainfall prediction using sea surface salinity. Journal of Climate, 34, 2473-2490. Reprint
Ummenhofer CC, Murty SA, Sprintall J, Lee T, and Abram NJ. (2021). Heat and freshwater changes in the Indian Ocean region. Nature Reviews Earth & Environment, 2, doi:10.1038/s43017-021-00192-6. Reprint
News Coverage
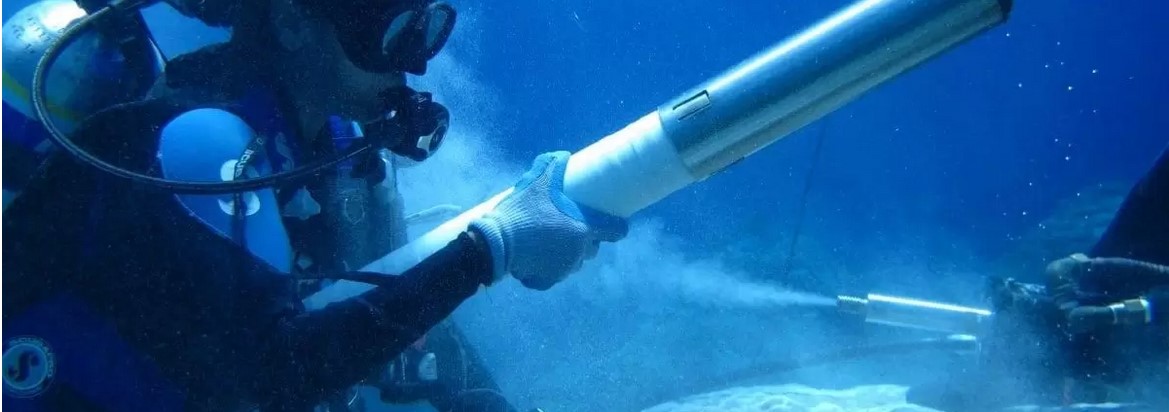
Scientists retrieve a coral core piece during an underwater drilling process. (Photo: Justin Ossolinski)
Scientists evaluate evidence for an intensifying Indian Ocean water cycle
Creating Synergy through art science
Saltier seas mean heavy summer rains for the US Midwest
Take weather prediction with a grain of salt and it gets better
Ocean salinity as a predictor of terrestrial precipitation